Impact of Space Data: “The Asteroseismic Revolution”
ESSC Panel Chair Saskia Hekker outlines how a shift in perspective can often lead to new insights. Much like how viewing a cathedral from different angles reveals its full grandeur, the transition to space-based telescopes has revolutionised our understanding of stars, unveiling new depths in the groundbreaking field of asteroseismology.
By Saskia Hekker
October 24, 2024
Recently, I had the pleasure of visiting the beautiful town of Cefalù in Sicily for a conference. This conference took place in the town hall, directly across from the cathedral. At first glance, the cathedral looked relatively small and modest to me, with two towers and a simple facade. It was only when we went inside and later saw it from the hill behind that we realised its true scale and beauty, with a stunning golden shrine: each perspective revealed something different, and all perspectives were needed to get the full picture.
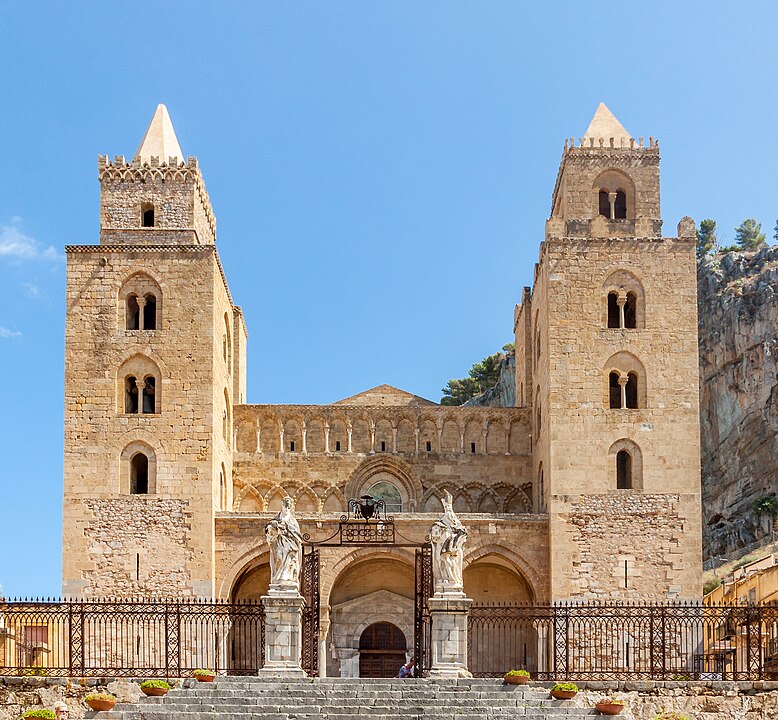
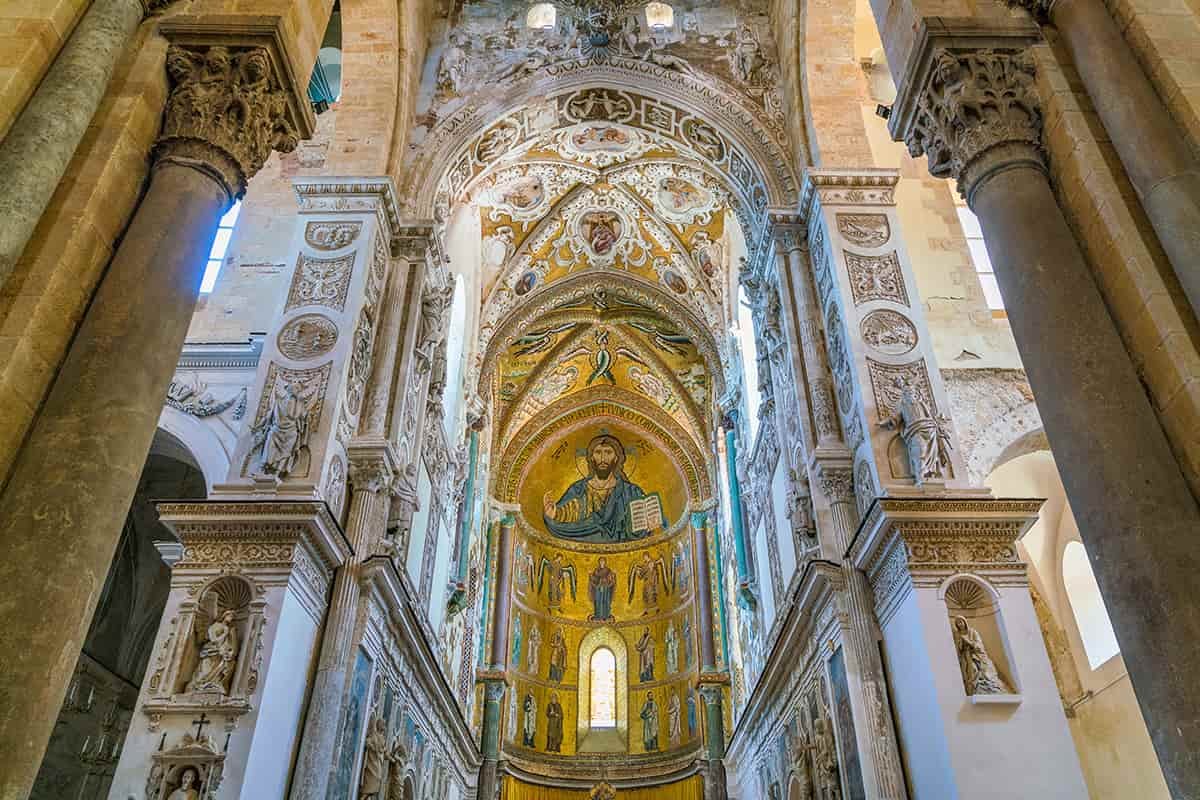
Taking this example more generally, viewing things from a totally different perspective, may allow us to see them in a different light – or discover entirely new aspects altogether. This shift in perspective has happened when we started to obtain timeseries observations of stars from space, leading to what we often refer to as the “asteroseismic revolution”.
Asteroseismology is the study of the internal structure of stars through their global oscillations. Similarly as for earthquakes, we can observe vibrations in stars. These vibrations are waves that travel with speeds that differ depending on the composition and the density of the material the wave travels through. This allows us to infer the properties of these materials and hence the internal structure of the Earth or stars.
For relatively cool stars (stars with similar surface temperatures as the Sun or cooler), theoretical computations predicted the presence of stellar vibrations resulting in oscillations with periods ranging from minutes to hours. These oscillations have small amplitudes of about 0.1-1% in brightness. In the early 2000’s, ground-based observing facilities became accurate enough to indeed observe these oscillations in a limited number of stars.
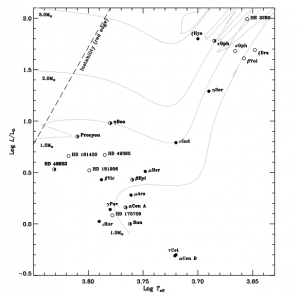
Observing stellar oscillations in cool stars requires many individual measurements taken in a relatively rapid sequence for a long time with as little interruptions as possible. The rapid sequence is needed to obtain several measurements per oscillation period, the length is needed to cover many periods of the oscillation and provide the resolution necessary to distinguish between different oscillations that are present. Interruptions in the observations, certainly periodic ones such as the day-night cycle on Earth, can lead to spurious signals that mimic oscillations and hamper the interpretation. Hence, these interruptions should be avoided.
Similarly as for seeing the cathedral from the inside and the hill behind it which gave a very different idea of its size and beauty, shifting to space-based telescopes has allowed us to see stars from a new perspective, and allowed us to discover entirely new phenomena. While primarily built to detect transiting exoplanets, asteroseismology has significantly, and in some cases dominantly, contributed to the success of space missions such as CoRoT and Kepler. These time domain surveys with very accurate photometry, high cadence of observations (i.e. a brightness measurement every minute or half hour) and near uninterrupted timeseries data have been a game-changer for observational asteroseismology.
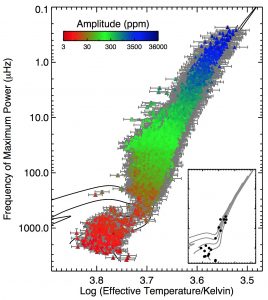
These space-based observations have opened an entirely new parameter space, leading to unexpected results in terms of:
- number of stars with detected oscillations (from a handful to tens of thousands);
- number of oscillations per star (from about 10 to 100);
- resolving power, i.e. the ability to distinguish between different oscillations.
This has opened a window to investigate the core of more than several stars as well as measure stellar rotation and magnetic fields – achievements that would have been impossible with ground-based facilities.
In addition to the specific stellar science enabled by these space missions, we have also learned several lessons about doing science more generally: The key takeaways from this are:
- going to a different parameter space pays off;
- scientific return can be maximised by embracing different analyses of similar data (“Someone’s noise is another one’s signal!”).
The next ESA mission that will contribute to the “asteroseismic revolution” will be the Plato mission. The primary goal of this mission is to find and characterise Earth-like exoplanets. The main advantage of Plato over previous missions is that brighter stars are targeted with high spatial resolution (not many stars end up on the same pixel) and for a relatively long time. Hence, entering different parameter spaces in terms of brightness and potentially resolving power (depending on the length of the observations of a particular field). In addition to the core program, Plato aims to maximise the scientific return by bringing together diverse fields of research with a broad and inclusive complementary science program. In this complementary program they coordinate any science that is not in the core program, welcoming contributions from the wider scientific community.
Most of the inferences we can nowadays make –and expect to expand on in the future– of the internal structures of cool stars have been made possible through the power of space science! Please join me in supporting ESA and other space agencies to continue to be able to build space missions that can move our scientific knowledge to the next level.